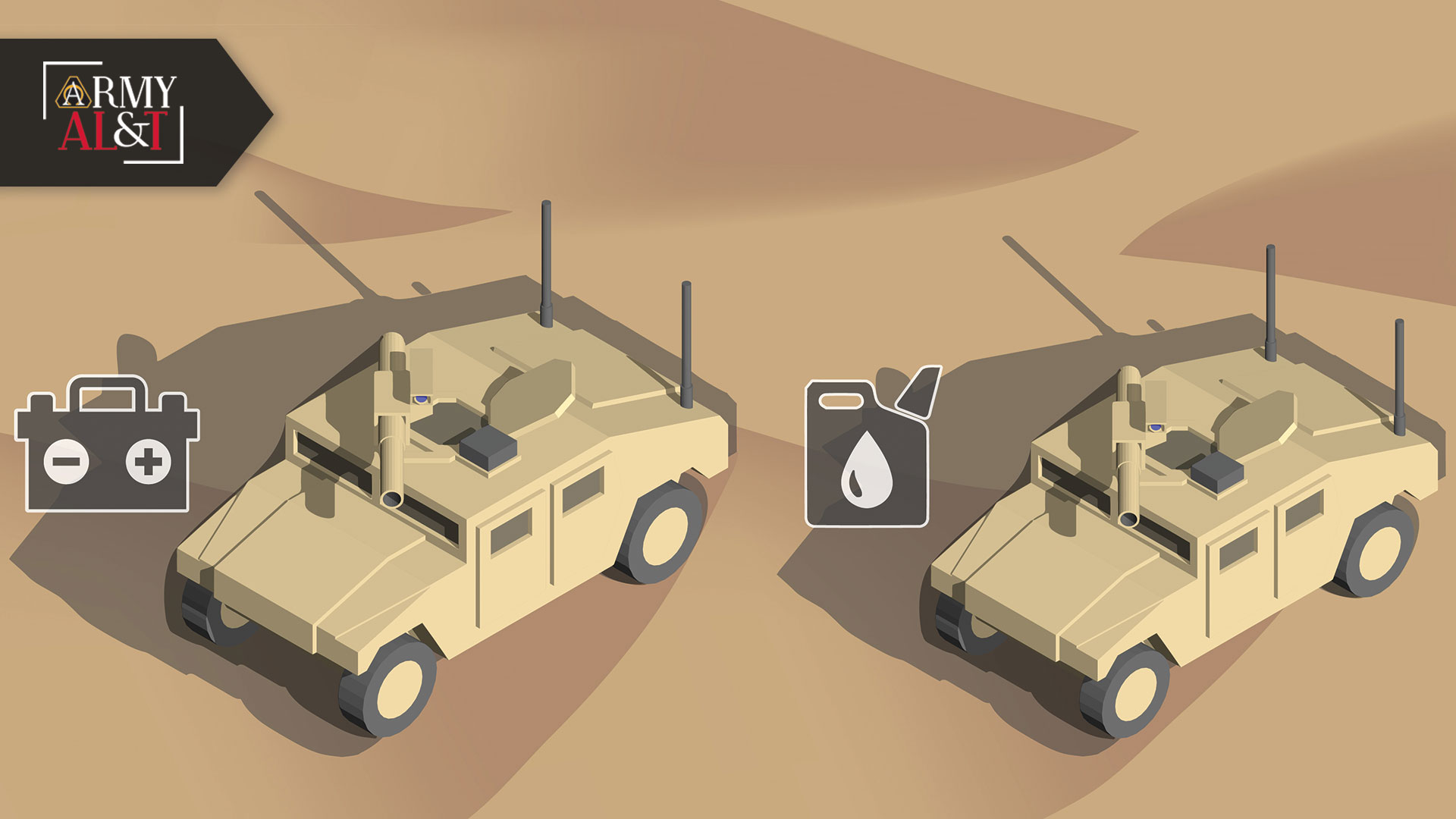
The Army’s requirement calls for the highest energy-density fuel combined with the lowest mass and volume—and gasoline and diesel win over alternative energy.
by Dr. David J. Gorsich and Dr. André Boehman
On any given day, a large Army division may use up to 6,000 gallons of fuel, and cost estimates to ship it to theaters of war in the Middle East can run as high as $400 per gallon. The Pentagon refers to this figure as the “fully burdened” cost of fuel because it incorporates a lot of factors that come into play when determining the cost.
In Afghanistan, for instance, the challenging geography and scant infrastructure, not to mention the ever-present roadside bombs, present logistical challenges that are expensive to overcome. Since there are no seaports, commercial trucks must transport fuel from ports in Pakistan and haul it overland. Once it reaches rearward areas, military transports must distribute the fuel to forward operating bases, many times by helicopter, which is the most expensive way to transport—hence the exorbitant costs.
In 2008 alone, approximately 68 million gallons of fuel were supplied per month to support U.S. military operations in Iraq and Afghanistan, and in the 2014 fiscal year, DOD consumed 87.4 million barrels of fuel, which supported its global presence, training at home and overseas, and logistical resupply. Obviously, reducing fuel consumption by military vehicles and generators, as well as developing other technologies to provide electricity at forward-deployed locations, would provide significant financial benefits and reduce casualties.
So why isn’t the U.S. Army using alternative forms of energy and powertrains more extensively to reduce fuel usage for its vehicles?
Tesla is building large semitrucks, and UPS and FedEx are starting to order these vehicles for delivery operations. It seems the entire automotive industry is migrating toward electrification as battery costs have dropped dramatically and recharge times and range have improved accordingly. With all of the major automobile manufacturers moving toward hybrids and electric vehicles, it’s easy to get confused and wonder why the Army is so far behind.
The reality is that the Army is not behind. It has experts in all of these fields who have been conducting research on alternative energy sources and hybrids for military vehicles for more than 20 years. In fact, the Army Rapid Capabilities and Critical Technologies Office recently awarded BAE Systems a $32 million prototype agreement to integrate a hybrid electric drive system onto a Bradley Fighting Vehicle. The development program is part of the Army’s effort to increase vehicle efficiency and boost power generation to support integration of future technologies and improve mobility for combat vehicles on the battlefield.
However, the bottom line is that there is a good reason the Army hasn’t unilaterally decided to switch to alternative fuels. The Army has a unique set of operational requirements, and no current fuel source meeting those requirements contains as much energy, by weight, as diesel or gasoline. Nor is there an alternative form of energy that can be carried or generated on board that, in terms of size or volume, is not at least four to 10 times that of gas and diesel. Electrification of the Army’s ground fleet (for example, by fielding integrated starter generators on tactical vehicles), is very important, but it still relies on diesel as the primary energy source.
This analysis focuses on energy density (the amount of energy stored in a system per unit of volume), conversion of that energy, as well as mass and volume requirements, to determine how they compare to current sources. The full technical paper was published in the Journal of Energy Resources Technology by Andrew Mansfield et al. Titled “Assessment of conventional and alternative energy carriers for use in military vehicle platforms,” the paper was published online Aug. 31, 2020.
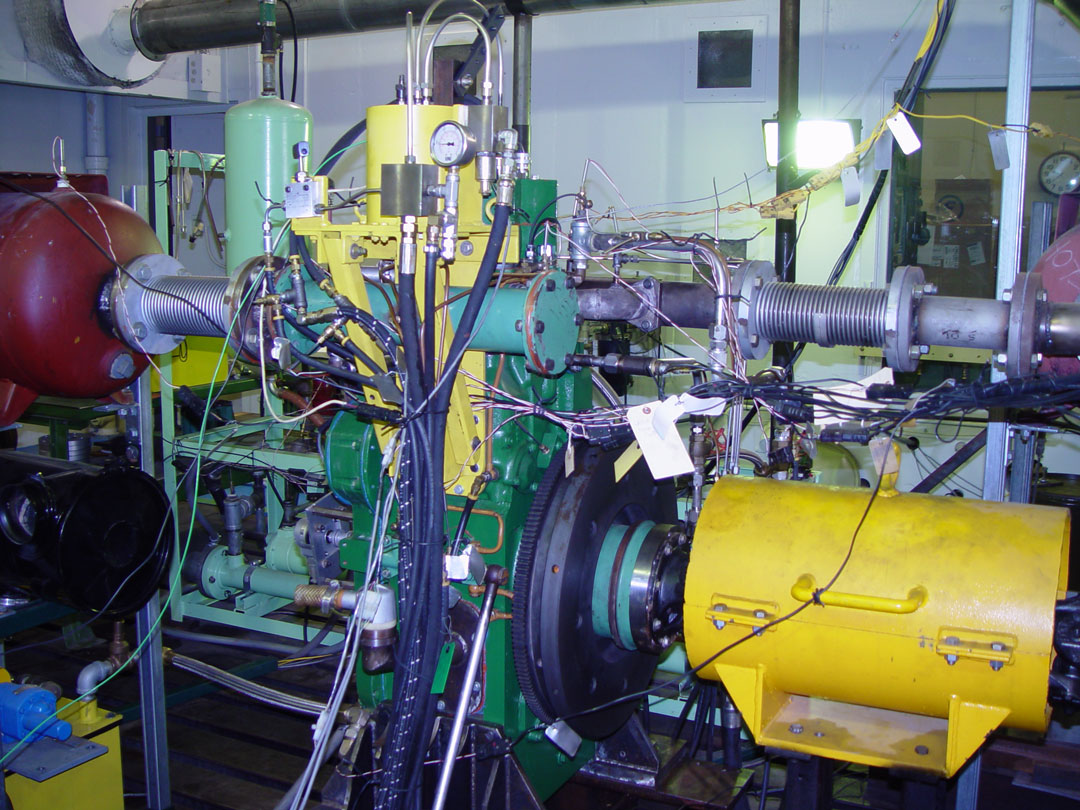
FLYWHEEL POWER: The flywheel of a Cummins engine, at lower right, is hooked up to a dynamometer, above, that measures its mechanical force, torque, or power—torque being a force that produces or tends to produce rotation or torsion. A flywheel is an energy carrier; it’s a heavy wheel attached to a rotating shaft so as to smooth out delivery of power. As it spins, the inertia of the flywheel opposes and moderates fluctuations in the speed of the engine and stores the excess energy for intermittent use. (Photo by Dr. Pete Schihl, U.S. Army Combat Capabilities Development Command Ground Vehicle Systems Center)
BACKGROUND
This study builds on past studies conducted primarily through the U.S. Army Hybrid-Electric Vehicle Experimentation and Assessment program. To understand why the Army still relies on diesel and gas, we need to understand the Army’s requirements, operating environment, and how energy is stored and transformed into vehicle motion.
That’s done through a process that starts with an energy carrier, which is a substance (fuel) or “material state” that stores energy that can be later converted to other forms such as mechanical work or power. We use the term “energy carrier” because it refers to energy that can exist in a variety of forms and can be converted from one form to another. Such carriers may include springs, flywheels, electrical batteries, pressurized air, hydrogen, petroleum, coal, wood and natural gas. A flywheel is a spinning mechanical device that is used to store rotational energy that provides continuous energy when the energy source, say torque from the engine, is intermittent.
Thus, energy carriers are the vehicle’s onboard store of energy, which is transformed into useful mechanical energy through a conversion device, such as combustion engines, electric motors or fuel cells. This process provides mechanical energy that can drive a vehicle transmission, which then can produce power such that:
Energy Carrier → Conversion Device → Mechanical Energy
There are several categories of transportation-applicable energy carriers, i.e., those that can reasonably be stored on board a vehicle, such as chemical and mechanical energy carriers. The various energy carriers and conversion devices considered in this analysis are illustrated in Figure 1, below. They are: hydrocarbon fuels (gas or diesel), batteries, supercapacitors, hydrogen (fuel cell) and flywheels.

THE OPTIONS FOR ENERGY: Vehicles can draw energy from a variety of sources, with conversion through a variety of devices. The graphic shows the energy carriers and conversion devices that the Army considers most relevant to fuel transportation, i.e., those that can reasonably be stored on board a vehicle. (Graphic by the authors)
Batteries and supercapacitors (electrochemical devices similar to batteries but designed to produce very high specific power, i.e. for use in regenerative brakes on hybrid vehicles) are often compared in terms of their energy and power. Batteries have a higher energy density (the amount of energy that the system can store), compared with supercapacitors, which have a higher power density (the rate of energy that the system can release).
That makes supercapacitors particularly suitable for storing and releasing large amounts of power relatively quickly, whereas batteries are capable of storing large amounts of energy over long periods of time.
ANALYSIS
Key military requirements assume that fuels will need to be transported to the battlefield, so there is a need for high-energy dense fuels with low weight, as well as a way to generate electricity. That’s because a typical Soldier carries four or five electronic devices—and in the future that burden will likely double—and such devices are useless without electrical power generation. Modern lithium ion (Li-ion) batteries cannot keep up with demand even if a Soldier could carry a large supply.
Figure 2 summarizes the performance characteristics of commercial energy carriers and promising future technologies, and illustrates the distinct superiority of diesel and gasoline fuels. They are by far the most widely used energy carriers and are used in conjunction with internal combustion engines or gas turbines with varying levels of commercialization.
Commercialization (COM) refers to the mass-market availability of a particular technology. Generally, the cheaper a technology is, the more widely available it will be for Army use. Therefore, the higher the commercialization ((↑) signifies increasing, and (↓) decreasing), the more practical the fuel is for implementation in Army vehicles. Subsequently, it becomes a factor in our best energy carrier options, and the new equation is as follows:
(Energy Carrier + Conversion Device) + (↑) COM = Mechanical Energy (Best Performance Characteristics)
Factors such as cost, reliability and commercialization strongly impact the usefulness of some of the options in Figure 2. Typical fuels (with their ease of commercialization given in parentheses), can be liquid, such as kerosene (high), diesel (high), gasoline (high) and ethanol (medium); or gaseous, such as methane and natural gas (medium to high). Lithium air (Li–air) batteries and hydrogen are middle to low performers, with similar energy density to fuels but an order-of-magnitude lower specific energy. Li-ion (medium to high) and nickel-metal hydride (Ni-MH; high) batteries, as well as flywheels (high) are at the lower end of performance with an order-of-magnitude lower energy density and specific energy values compared with high-performance electrochemical energy carriers.

HOW THEY PERFORM: An analysis comparing the performance of various possible energy carriers for Army vehicles, including commercial energy carriers and promising future technologies, shows a marked superiority for diesel and gasoline fuels. Specific energy is energy per unit of mass; energy density is the amount of energy stored in a battery or tank per unit of volume. (Graphic by the authors)
Ni-MH batteries have become very well established in the marketplace, while Li-ion batteries are more recently commercialized. Li-air batteries (low) are a very promising future technology that is not yet commercial.
Many full or partially electric vehicles from the past decade use Ni-MH batteries. Newer electric automotive vehicle manufacturers, such as Tesla, have chosen to use Li-ion batteries.
The conversion devices considered were combustion engines, electric motors, and fuel cells. Their performance characteristics can be seen in Figure 3, which shows a measurement of the actual performance of each power source versus its power output. Electric motors have the highest level of performance, but, as we saw earlier, they are connected to batteries that don’t have enough stored energy to last for military applications. Gasoline and diesel combustion engines are next, with methane engines at the lowest level.
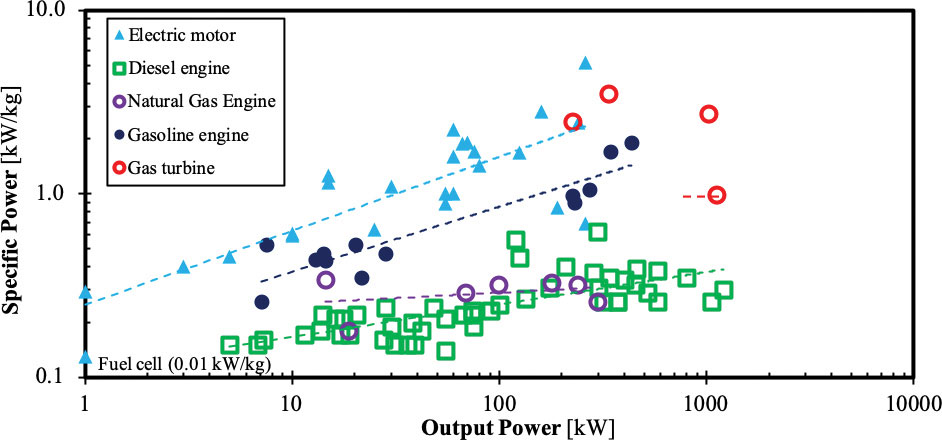
THE QUESTION OF CONVERSION: A comparison of various conversion devices—combustion engines, electric motors and fuel cells—looked at the actual performance of each power source versus its power output. Although electric motors have the highest level of performance, the authors found, they are connected to batteries that don’t have enough stored energy to last for military use. Specific power, or power-to-weight ratio, is a measure of engine performance in units of kilowatts (kW) per kilogram (kg). (Graphic by the authors)
ENERGY CARRIERS TAKE UP SPACE
Another important factor when considering alternative energy carriers is how much vehicle space will be required to transport the requisite fuel as well as all of the ancillary equipment necessary to operate and adequately power the vehicle system.
In order to assess and compare approximately how much onboard space each alternative energy carrier would require, researchers evaluated the approximate space claims by comparing the energy carrier volume and powertrain mass associated with each carrier with regard to the High Mobility Multipurpose Wheeled Vehicle (HMMWV) and Family of Medium Tactical Vehicles (FMTV) platforms. Figure 4 illustrates the predicted total powertrain mass and energy carrier volume for various single and dual hybrid energy carriers for the two vehicle systems. The results indicate that diesel and gasoline fuels are clearly the optimal energy carriers for both vehicle systems, with the lowest total powertrain masses and low energy carrier volumes.
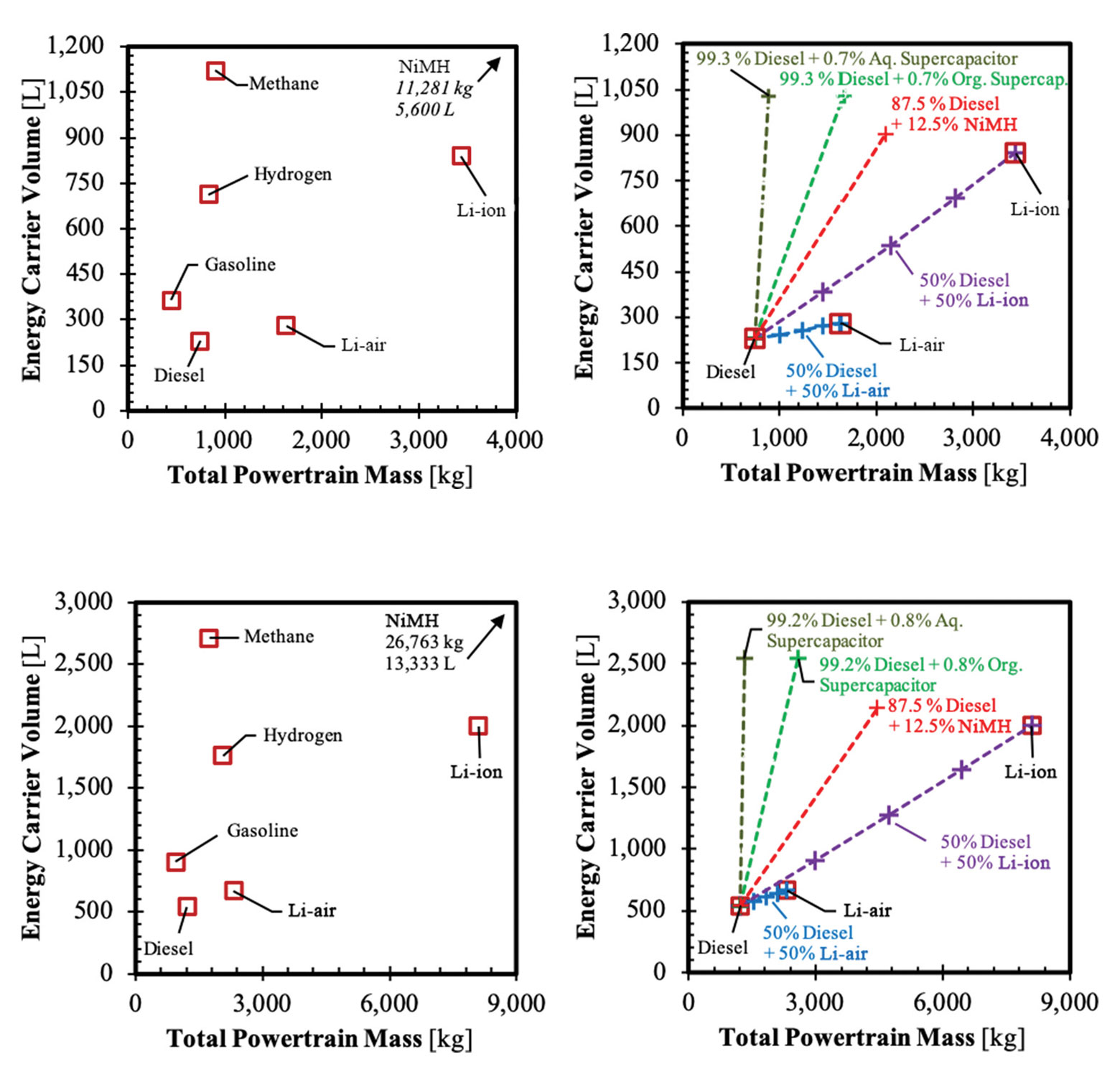
TAKING UP SPACE: The amount of vehicle space required to transport a fuel, in addition to the equipment necessary to operate and power the vehicle system, is a key consideration when considering energy carriers. The authors’ analysis looked at the approximate space claims of each energy source by comparing the energy carrier volume (in liters) and powertrain mass (in kilograms), specifically for the Humvee and FMTV platforms. The graphic at left is for various single-energy carriers; at right is a comparison of dual hybrid energy carriers, e.g., diesel + battery or supercapacitor. The results indicate that diesel and gasoline fuels are optimal for both vehicle systems. (Graphic by the authors)
This is true of the results for both single energy carriers in the left-side graphs of Figure 4 and for dual hybrid carriers (diesel + battery or supercapacitor) in the right-side graphs. Of the dual hybrid energy carriers, diesel fuel + Li-air hybrid is clearly optimal, yielding the greatest rate of hybridization (50 percent diesel and 50 percent Li-air) considering the relatively small mass and volume increases. These results also clearly indicate the dramatic benefits of Li-ion and Li-air battery technology over more traditionally used Ni-MH.
A prime candidate for alternative-energy carrier for military vehicles would include an optimized fuel source and conversion device, a high level of commercialization, as well as a system mass and volume as small as possible. In general, smaller (↓) mass and volume (M&V) alternatives increase the operational effectiveness of vehicles and, therefore, become part of our equation as follows:
(Energy Carrier → Conversion Device) + (↑) COM + (↓) M&V = Mechanical Energy (Best Performance Characteristics)
With respect to batteries in both single and dual energy systems, the three alternative energy technologies considered here resulted in dramatically different total powertrain mass and energy carrier volumes—much larger than for diesel fuel systems. Use of Ni-MH batteries results in a total mass 15 to 22 times larger and energy carrier volume 25 times larger than the diesel fuel system. Li-ion batteries are a dramatic improvement over Ni-MH, resulting in a total mass only 4.5 to 7 times larger and energy carrier volume 4 times larger than the diesel fuel system.
Even with this improvement, the large mass and volume increase over a diesel fuel system presents a significant challenge to its implementation, as driving performance and usable volume would likely fall dramatically. The effect of large mass and volume increases for Li-ion battery systems in similar large vehicles is evident in the short 300 – 500 mile range of the Tesla semitruck, which uses Li-ion batteries, compared with a more typical 1,000-mile range for a diesel semitruck.
Relative to diesel fuel, both hydrogen and methane yield similar total powertrain masses but significantly larger (3-5 times) energy carrier volumes. This highlights the primary issue with the low energy density of gaseous fuels and indicates that at existing and near-term technology levels, if these fuels are used as the single energy carrier for military ground vehicles, a large portion of their usable payload carrying capacity (volume) would be lost.
CONCLUSION
The Army has a unique set of requirements that cannot currently be better satisfied with anything other than gas or diesel as the primary energy carrier. Considering the high torque performance of diesel combustion engines, diesel fuel is clearly the optimal energy carrier for the Army’s ground vehicle platforms, from the perspective of both the total powertrain mass (energy carrier + conversion device), and stored energy volume.
Military applications provide common and unique challenges for energy storage systems and energy density, with regard to mass and volume, and are critical challenges for commercial and military energy storage systems. Energy storage systems for military applications must be able to be stored and operate reliably at low and high temperatures (minus 46 to 88 Celsius) and under greater shock and vibration conditions than commercial systems. Cooling systems for military energy storage and export power solutions are complicated by the harsh environmental conditions they must withstand. Consequently, this combined scenario continues to drive the Army toward liquid fuels and their physical properties.
In the future, for the Army to consider a fleetwide, wholesale change that moves away from hydro carbon fuels, it needs to develop a very detailed strategy and step-by-step paths to acquire any new technologies. This hasn’t happened yet because there are still too many obstacles that need to be resolved before alternative energy carriers can become a viable option for full-scale use. So for now, the Army still relies on carbon-based fuels, which will likely remain the primary fuel. It is unlikely, in terms of scale and scope, that the Army will transform to something else in the next 10 years. While alternative power-generation exists now in many different forms, alternative fuels aren’t ready for prime time when it comes to large-scale Army use.
For more information, visit https://arc.engin.umich.edu/, the website of the Automotive Research Center, a university-based U.S. Army Center of Excellence for modeling and simulation of military and civilian ground vehicle system. Co-authors Gorsich and Boehman are the center’s government executive director and leader of its engines work group, respectively. Or contact Gorsich, Chief Scientist, U.S. Army AFC-CCDC GVSC, 6501 E. 11 Mile Road, Building 200A, Warren, MI 48397-5000; or david.j.gorsich.civ@mail.mil.
- DAVID J. GORSICH serves as the Army’s chief scientist for ground vehicle systems. His current research interests are vehicle dynamics and structural analysis, reliability-based design optimization, underbody blast modeling, terrain modeling and spatial statistics. He holds a Ph.D. in applied mathematics from the Massachusetts Institute of Technology, an M.S. in applied mathematics from George Washington University and a B.S. in electrical engineering from Lawrence Technological University. Previously he served as the U.S. Army Tank Automotive Research, Development and Engineering Center’s (TARDEC) associate director for modeling and simulation, culminating his service at TARDEC, where he was also the acting director for strategic plans and programs (G5) and the team leader for robotics and vehicle intelligence. He also served the U.S. Army Materiel Command, the Army Research Laboratory and the Assistant Secretary of the Army for Acquisitions, Logistics and Technology.
- ANDRÉ BOEHMAN is a professor of mechanical engineering at the University of Michigan, director of the university’s Walter E. Lay Automotive Laboratory and the associate director of the Automotive Research Center. He holds a Ph.D. and an M.S. in mechanical engineering from Stanford University, and a B.S. from the University of Dayton, all in mechanical engineering. His research interests are in alternative and reformulated fuels, combustion and pollution control. He served as editor-in-chief of the Journal of Fuel Processing Technology and presently is an associate editor for Energy & Fuels. He was elected a 2011 Fellow of the Society of Automotive Engineers. He has received the 2009 John Johnson Award for Outstanding Research in Diesel Engines and the 2009 Arch T. Colwell Merit Award from the Society of Automotive Engineers.
Read the full article in the Fall 2020 issue of Army AL&T magazine.
Subscribe to Army AL&T News – the premier online news source for the Army Acquisition Workforce. Subscribe