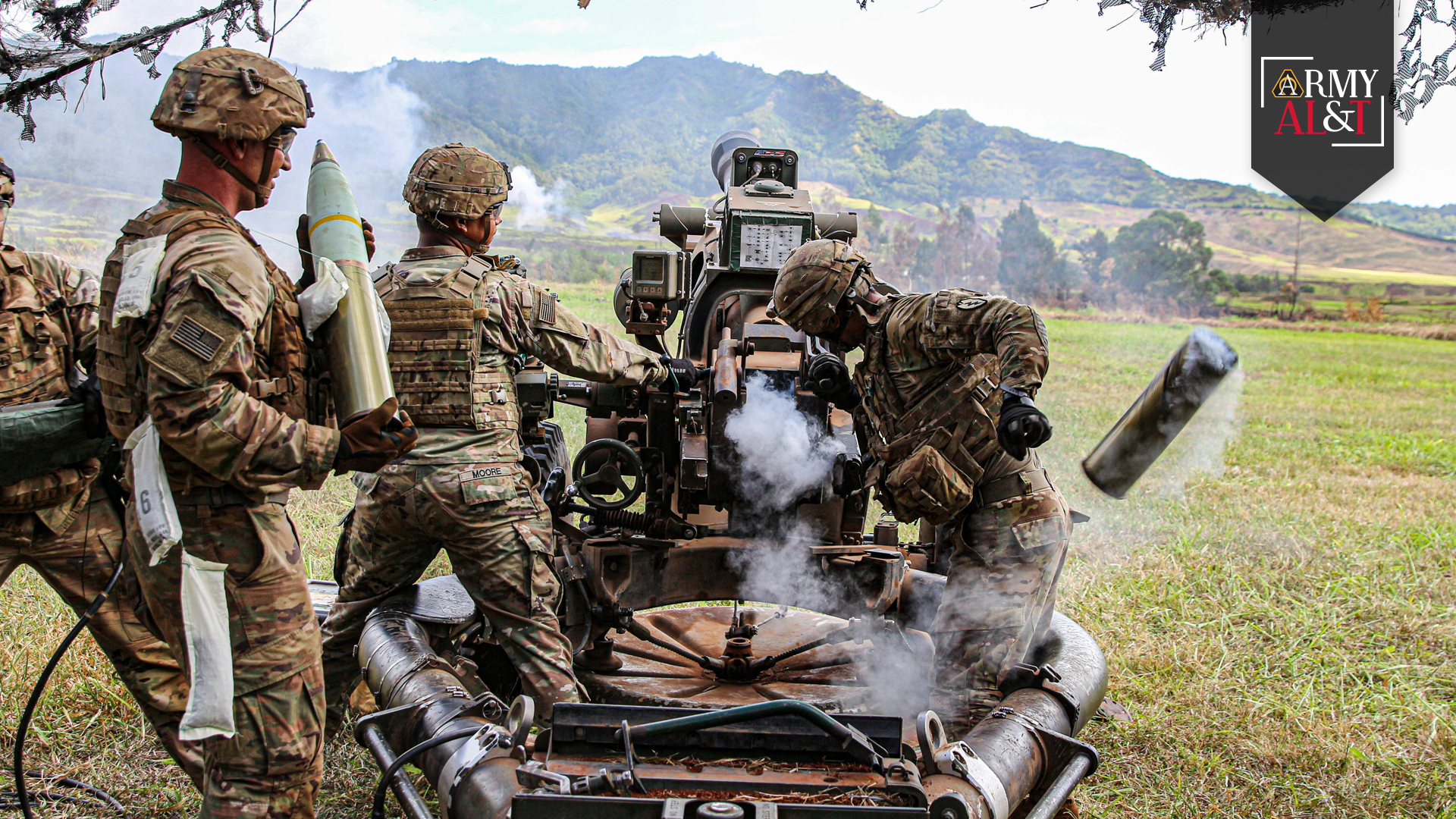
CANNON UPGRADES: Previously, cannon and propulsion technologies have mostly been upgraded and developed on separate development paths leveraging prior legacy designs. PD TAS has initiated a project to develop a new cannon system that can support future capability upgrades such as increasing the systems rate of fire while improving reliability and safety. (Photo by 1st Lt. Stephanie Snyder, 25th Infantry Division)
A team of engineers and statisticians take a systems approach to developing cannon artillery.
by Peter Harvey
A search in the Defense Technical Information Center database for technical terms like ignition, artillery, propulsion design or cannon design will result in reports from the 1950s through the 1970s written by very knowledgeable people providing a wealth of information on a particular topic. What may be missing from these reports is the “system interface” approach.
In this context, a “system” is defined as a group of subsystems or components that are assembled to create a functioning artillery howitzer. In terms of interfaces (i.e., how one subsystem works with another) that make up a complete artillery system, the cannon-ammunition interface can arguably be the most important. Through the progression of artillery design spanning over decades, cannon technologies as well as propulsion technologies have been upgraded and developed, mostly on separate development paths leveraging prior legacy designs.
This lack of a systems approach is a likely contributor to issues of poor reliability, lack of interoperability, unoptimized performance and safety concerns. By taking advantage of advancements in modeling and simulation in both cannon and propulsion design, as well as adding a heavier presence of statistical modeling and analysis, we have an opportunity to provide a fresh look at developing a robust cannon-ammunition interface.
IT’S ALL ABOUT THE TEAM
In 2022, Project Director for Tactical Artillery Systems (PD TAS) initiated a project to develop a new cannon system that can support future capability upgrades, such as increasing the systems rate of fire while improving reliability and safety. Eager to begin, PD TAS created a team with experts from across the U.S. Army Combat Capabilities Development Command (DEVCOM) Armaments Center, DEVCOM Army Research Laboratory, Program Manager Combat Ammunition System and the Army Test and Evaluation Command in an effort to assemble a group of engineers and statisticians from multiple artillery competencies, each representing a piece of the cannon-ammunition interface. Once assembled, the work began.
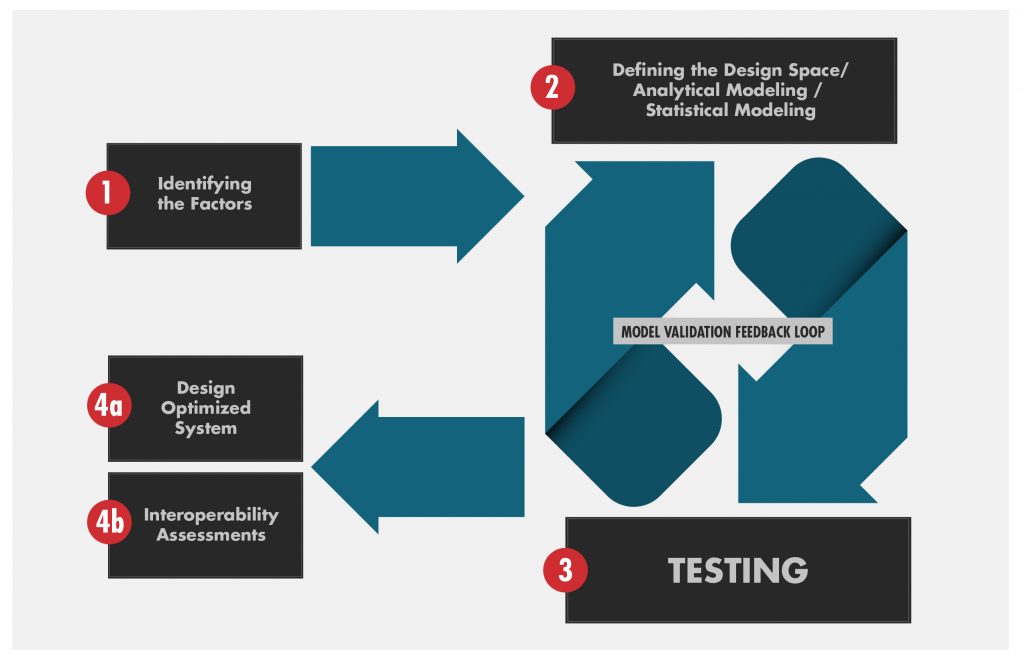
DESIGN OF EXPERIMENTS: Overview of the design of experiments process created to design the cannon and propelling charge and the interface between the two. (Graphic by Peter Harvey, PD TAS)
DIGITAL SYSTEM DESIGN TOOL
The diverse team held many discussions and brainstorming sessions to develop a plan of action. Through collaborative discussions and experiences, the team agreed that the focus of this cannon design should be on ignition, with the premise that improved ignition will create an environment to develop future complex systems that can survive in the battlefield with higher reliability.
With the focus established, the team then defined “improved ignition.” For this effort, the team defined the term as a reduction in the rate of change in pressurization over the change in time during the ballistic cycle as well as a reduction in pressure waves while maintaining established projectile muzzle velocity performance.
This project employed the statistical tool called design of experiments. This is a novel approach in the design of both the cannon and the propelling charge as well as the interface between them.
An extensive design of experiments was used in conjunction with other statistical modeling tools and existing interior ballistic models. In addition to the models, the team obtained test data from a ballistic simulator, which is a test apparatus that ignites propellant in a clear tube to allow for the visual analysis of the ignition phase of the ballistic cycle. The ballistic simulator fixture is also equipped with the capability to capture pressure traces at both the simulated breech end and the simulated projectile base locations. Using such tools and incorporating them into the design of experiment process makes it possible to identify the strong relationships among different factors—or, in other words, to mathematically understand and identify how different features of the cannon design affect different features of the propulsion design, and vice versa.
Identifying all these features, also known as “factors,” was the backbone of this process. After the team defined all the factors (e.g., dimensions, weights and rates, as well as other specifications and characteristics) between the cannon and propulsion systems, a range of values for each factor was determined. From this, the team was able to produce thousands of different design configurations. Then, using the interior ballistic modeling tools, the team was able to predict the performance of each configuration. The statistical analysis combined all these predictions to produce a surrogate model, later named the Digital System Design Tool (DSDT), which enabled the ranking of all the factors in influential order.
A system engineering tool called “value functions,” which provides a way to define acceptability and to rank the importance of different characteristics, was then applied, giving the team the criteria necessary to determine good configurations that will improve ignition as well as configurations that do not.
Validation is the key to any modeling effort, as it gives the effort credibility by confirming outputs through actual testing. Validation also enables modeling enhancements that will increase its fidelity. For the DSDT effort, ballistic simulator testing was the best tool available to assess ignition. The DSDT provided thousands of viable cannon configurations that could improve ignition of the propelling charge. The team chose several of these configurations for testing in the ballistic simulator to provide data for the model validation process. The team used this data to update the models.
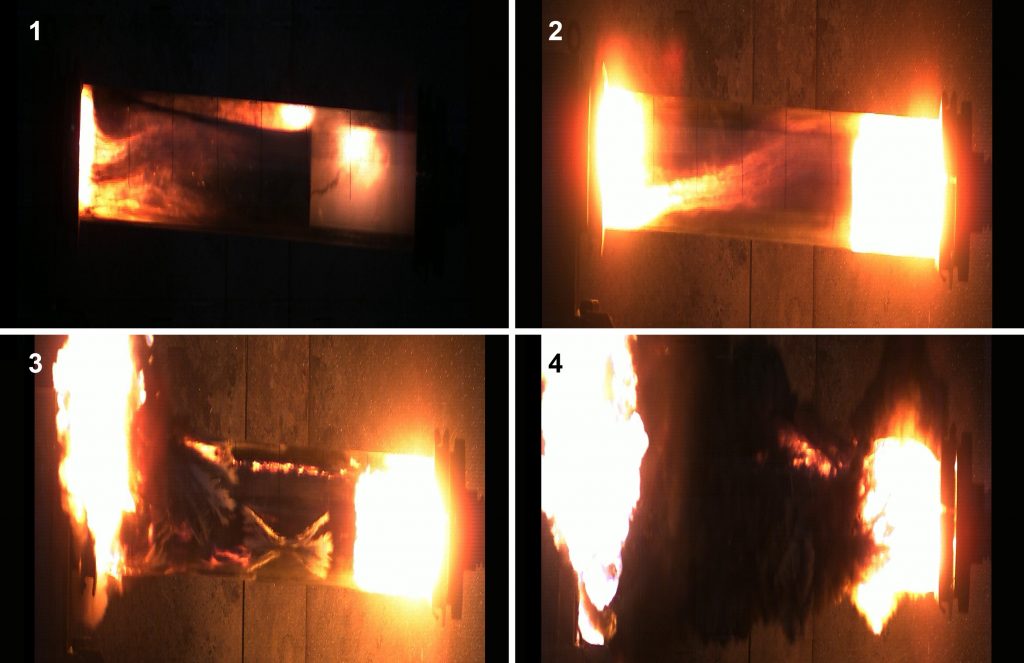
SIMULATOR IGNITION: Screen shots from a high-speed video shows ignition in a ballistic simulator during a test event in November 2023 at Yuma Proving Ground, Arizona. The ballistic simulator is a test apparatus that ignites propellant in a clear tube to facilitate for visual analysis. (Image by Peter Harvey, PD TAS)
PULLING IT ALL TOGETHER
At this point of the project, the team was in place, the tools were created and validated, and there were thousands of different cannon configurations to potentially improve ignition to be evaluated.
The next step in this process was to use the surrogate model to navigate through all the cannon design configurations. The team filtered the cannon configurations using the value functions that consider other factors that are important to the design of cannon artillery. This includes but is not limited to projectile muzzle velocity, cannon chamber pressure limits and manufacturing capability. Once all these other factors were included, the team was able to successfully down-select to a single cannon configuration that will improve ignition. A complete cannon is being manufactured to this configuration and is scheduled to be live-fire tested late in the third quarter of fiscal year 2024 as the final validation of the team’s efforts.
CONCLUSION
In addition to developing a new cannon chamber to improve ignition, this project brought together a technically diverse team to develop a novel DSDT for a collaborative design of the cannon-propelling charge interface, while keeping focus on the system aspect. The tools and processes developed allow team members to conduct numerous analyses, as required, without the need for timely and costly live-fire testing. The team can use these modeling and simulation tools to develop whole cannon and propelling charge systems jointly or even assess the interaction, of either developmental propelling charges in an existing cannon or a developmental cannon with existing propelling charge systems.
One major potential application of the DSDT can be applied to the conflict in Eastern Europe. This tool can provide an assessment of interoperability among the many different artillery cannons and propellant system combinations currently seen. Not knowing how these systems interface can lead to catastrophic results. This is also a major benefit to the U.S. Army as it can all be completed without the extra cost and scheduling implications of traditional prototyping and live-fire development procedures.
For more information, contact the author at peter.j.harvey.civ@army.mil.
PETER HARVEY is a program management engineer with Project Director Tactical Artillery Systems at Picatinny Arsenal, New Jersey. He holds a B.S. in mechanical engineering technology from University of New York at Alfred. He is a DAWIA certified Practitioner in engineering and technical management.